Numerous scientific studies have shown that air inside commercial and residential buildings is contaminated by a mixture of hundreds of gaseous pollutants and particulate matter. These contaminants may be present in concentrations sufficient to adversely affect the health of inhabitants. In addition to potentially high concentrations of indoor pollutants, the indoor exposures are significant and may vary from approximately eight hours/day to 16-24 hours/day for commercial buildings and residences, respectively.
Indoor quality problems associated with residential homes differ from nonindustrial, commercial buildings. Commercial and institutional buildings are generally equipped with automatically controlled ventilation systems, which can reduce contaminant concentration by diluting indoor air with less contaminated outdoor air. In residential buildings, the air exchange occurs by natural ventilation (open windows and doors) and by infiltration. Only a small percentage of U.S. households are equipped with the energy recovery system (fresh air systems) which provide a continuous supply of fresh air.
Ventilation together with air filtration are important methods for reducing concentration of airborne contaminants. Higher ventilation rates can improve the indoor air quality; however, the amount of energy needed to condition the indoor air in cold and hot/humid environments could be significant. On the other hand, under-ventilation may have a negative impact on the quality of indoor air, but at lower energy cost. To avoid over-ventilation (wasted energy) as well as under-ventilation (potential for poor air quality), a cost-effective ventilation control strategy is needed, such as demand-controlled ventilation (DCV).
Measurement and control of indoor CO2 concentration has been widely used as an indicator of human-related contaminant sources to control DCV systems, however, there are challenges associated with this approach. The main issue with the CO2 based DCV systems is the absence of information on the concentration of indoor gas phase contaminants and particular matter (PM), which could reach hazardous levels. Continuous emission of volatile organic compounds (VOC) from building materials, furniture, carpets and released during human activities such as cooking, cleaning, or smoking cannot be detected with a CO2 sensor. The same situation exists with measuring the PM concentration during human activities such as cooking, smoking, and cleaning.
To address the above challenges, an array of gas and PM sensors could be used to measure concentration of selected indoor contaminants and to control the ventilation rate. In a residential application, the same sensor system could control the residential HVAC, fresh air system as well as range hood exhaust. The benefits of sensor based IAQ control becomes clear in indoor spaces where changes of CO2 concentration are too small for DCV system to be activated, but the concentration of other indoor air pollutants is high.
What Pollutant Should Be Measured?
In indoor environments hundreds of chemical compounds (VOC and inorganic), bioaerosols and PM can be found, however, it is not practical to effectively implement sensors to measure low concentrations of even a small percentage of the indoor air pollutants. One approach to this issue is to identify pollutants that are known to have an adverse effect on human health, and based on this identification to select appropriate sensors. The schematic process is shown below.
The selection of the most hazardous pollutants is based on the measurements of indoor concentrations, human exposure and health effects. The World Health Organization (WHO) has identified the following pollutants as being of most concern: benzene, carbon monoxide, formaldehyde, naphthalene, nitrogen dioxide, PM2.5, polycyclic aromatic hydrocarbon (PAH), radon and trichloroethylene.[1]
Based on measurements of the concentration of indoor pollutants in U.S. residences and the assessment of associated health hazards, a following list of nine pollutants was generated: acetaldehyde, acrolein, benzene, 1,3-butadiene, 1,4-dichlorobenzene, formaldehyde, naphthalene, nitrogen dioxide and PM2.5.[2]When a different metric that took into consideration the cost associated with exposure to the indoor air pollutants, such as Disability Adjusted Life Years (DALYs), the list of the top five pollutants of most concern included: PM2.5, SHS (second hand smoke), radon, formaldehyde and acrolein.
Based on the above studies and identification of the most hazardous indoor air pollutants, it is not a simple task to select the appropriate sensors. Sensors to measure PM2.5, formaldehyde, nitrogen dioxide and VOCs should be considered as candidates for smart, demand-controlled ventilation systems. Also, countries with different lifestyles and environmental conditions could have a different list of major pollutants and different Exposure Limit Values (ELVs) that will need to be considered for a global market.
Low-Cost Sensors
During the last decade the improvement of existing technologies of PM and gas sensors has allowed the introduction of various types of low-cost sensors for air pollution monitoring. The majority of commercial low-cost sensors are based on the following principles of operations:
- PM sensor – light scattering
- Photo-ionization (PID) – UV light
- Electrochemical sensors – amperometric or potentiometric type
- Metal oxide sensors (MOx) – resistivity change
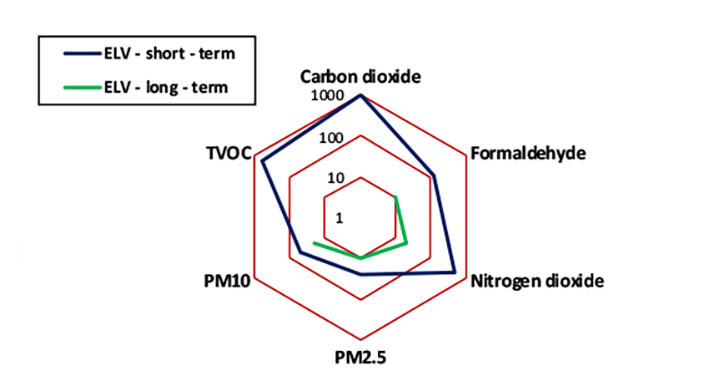
One of the critical sensor parameters is the low-level concentration detection limit. These limits of detection should be lower than the Exposure Limit Values (ELV) for specific pollutants.[3] Figure 1 shows the short- and long-term ELV for selected pollutants. For example, the long-term ELV for formaldehyde is 9 µg/m3 and for PM2.5 it is 10 µg/m3. Sensors should be capable of measuring these concentration levels. Benzene as a one of the most critical, human carcinogenic pollutant has the long-term ELV = 3 µg/m3 per U.S. guideline and this is a significant challenge for low-cost sensors. It is recommended to reduce indoor exposure levels for benzene as low as possible. [1]
PM sensors. The reported performance of low-cost particle sensors across the literature is somewhat mixed, depending on the type of particle sensor and method of evaluation. There is a wide range of commercially available low-cost PM sensors which employ a light-scattering technique. These can typically detect particles with aerodynamic diameters ranging from 0.3 µm to 10 µm, and they transform the measured signal into a mass concentration. The limit of detection of low-cost PM sensors is typically between 5 to 10 µg/m3. The main drawback of using these sensors is the data quality, which may be sensitive to interference from environmental conditions, limited reproducibility, drift over time and the composition of the particles. If the aerosol being measured exhibits a different refractive index, density, shape factor or size distribution than the aerosol used for calibration, the measured concentration could be affected by these differences. Figure 2 illustrates this, it shows the measured concentration of cigarette smoke by two sensors, one calibrated with cigarette smoke and another one with different aerosol.
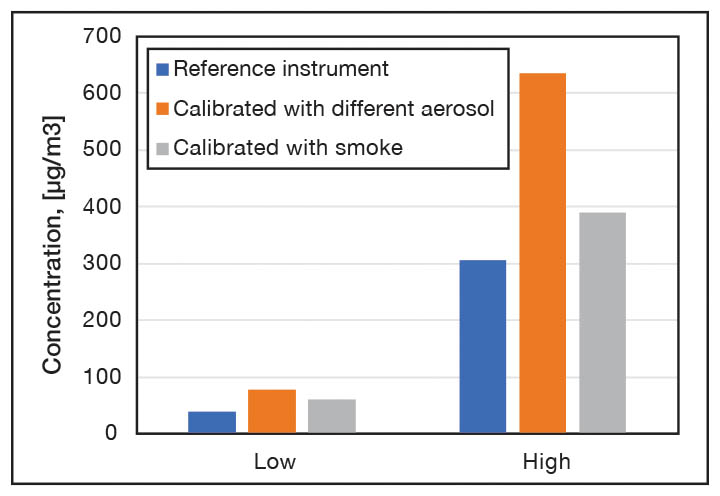
Formaldehyde sensors. Sensors used for measuring the formaldehyde concentration can be based on several principles, however, the most popular and feasible are the electrochemical sensors. They operate by reacting (oxidation – reduction) with the gas of interest and producing an electrical signal, which is proportional to the gas concentration. Since a current is generated in the process, the electrochemical sensors are often described as an amperometric type sensor.
Typical electrochemical sensors consist of a hydrophobic membrane, working electrode, counter electrode and layer of electrolyte. Despite their similar appearance, there are many different ways that electrochemical sensors are constructed. Their performance could be significantly different for each of these sensors, in terms of accuracy, sensitivity, selectivity, cross sensitivity, response time, and life expectancy. Detection limits depends on the structure of sensor and, typically, is in the range of 30 – 50 µg/m3. Temperature and humidity dependency as well as cross sensitivity are the major challenges affecting sensor accuracy. Figure 3 shows the response of two different electrochemical sensors to formaldehyde at different humidity levels.
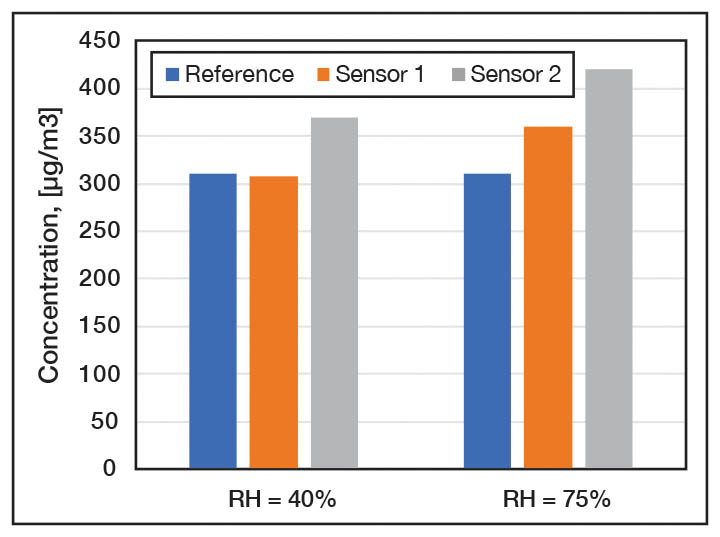
VOC sensors. Electrochemical and semiconductor methods are the two most widely utilized gas sensing mechanisms. Metal oxide semiconductor (MOS) sensors are a resistive type sensors, since the resistance changes upon interaction with target gases. To enhance the sensor reactivity, the temperature of the sensing material is increased by using a local heater. The gas concentration is detected by measuring the resistance of MOS gas sensor. The most commonly used (MOS) gas sensors employ ZnO and SnO2 because of the good gas response, compact size and low cost. They are widely used to the measure concentration of VOCs in industrial applications. The temperature and humidity impact on sensor accuracy as well as the cross sensitivity are the major drawbacks. The presence of hydrogen sulfide, ammonia and alcohol in ambient air also strongly affects the accuracy of the MOS sensor. Figure 4 illustrates the impact of relative humidity on VOC measurement.
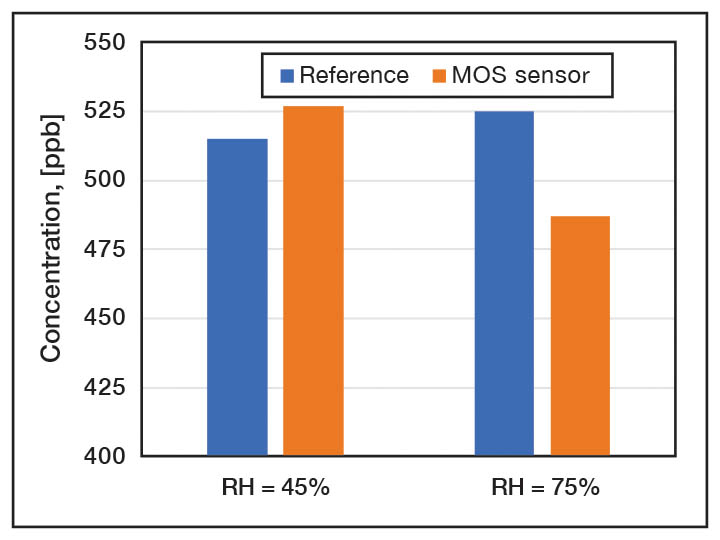
Sensor Input
The main challenge with the measured concentration of indoor air pollutants is the creation of a metric which could be used to communicate with the demand – controlled ventilation system. One approach is the direct comparison of the measured concentration values (C) to the ELV for the specific pollutants.
When: Ci[µg/m3] > ELVi or Ci[µg/m3]/ ELVi > 1
activate ventilation system, fresh air or range hood exhaust
For example, the algorithm will trigger activation when measured concentration of PM2.5 is greater than the ELV = 10 µg/m3. Another approach is to create a simple multipollutant index (X) by combining all measured pollutants, similar to the Indoor Air Quality Index concept.
X = ∑ Xi = ∑ λi (C/ELV)i = λ1(C/ELV)1 + λ2(C/ELV)2 + λ3(C/ELV)3 + …
where: λ is the weighting factor assigned based on priority with ∑λi = 1
i – specific pollutant such as PM2.5, formaldehyde, CO2
The simple, algebraic sum is an elegant way to include inputs from all sensors, however this may not be the optimal way to describe the interaction effects among the various pollutants. Interaction between pollutants may result in health effects that may be additive, synergistic, or antagonistic. The additive effect can happen when the exposure to several pollutants produces an effect equal to the sum of the effects of individual pollutants. Synergistic effects are the most challenging cases, since the total health effect is greater than the sum of the effects of each acting alone.
Conclusion
Low-cost particle and gas sensors are an attractive addition to the demand-controlled ventilation systems and, potentially, for future applications such as residential fresh air systems and/or range hood exhausts. Despite all the limitations, such as cross sensitivity issues, inadequate limits of detection or the impact of environmental conditions, low-cost sensors can play an important role in IAQ application.
The majority of current portable air cleaners are equipped with at least one sensor, or array of sensors. These sensors not only can measure and display the pollutant concentration, but also trigger an action such as changing flow rate, and potentially, to detect the state of filtration system. Even if the triggered action occurs at a different concentration than the ELV, the benefits are evident. As an example, we can look at the cooking events when the PM2.5 concentrations can exceed 1000 µg/m3. Due to higher detection limit, PM sensors could activate a cleaning system or exhaust fan at higher concentration that the recommended ELV = 10 µg/m3, but the exposure to high concentration will be significantly reduced.
Low-cost air pollution sensors can also be incorporated into more complex monitors, which can provide users with useful information regarding concentration levels of some air pollutants, and may help them to take actions to improve indoor air quality.
References:
1. WHO guideline for indoor air quality: selected pollutants, 2010.
2. JM Logue at el., Hazard assessment of chemical air contaminants measured in residences, Indoor Air, 2011.
3. AIVC Contributed Report, 2017.